Magnetisation Dynamics
The understanding and control of the magnetization dynamics in ferromagnetic thin films and nanostructures is important for future applications in spintronics. When an ultrashort laser pulse (at the femtosecond time scale) interacts with a ferromagnetic material, the magnetic moment of a system can be quenched very rapidly. This is referred to as ultrafast demagnetization and there have been many experimental and theoretical works aimed at explaining the underlying mechanisms. At longer times (nanosecond time scale), precession of the magnetization is observed, and the detailed magnetization dynamics depends on the demagnetization field that results from patterning a thin film system into sub-1 μm elements.
We can characterize the magnetization dynamics of ferromagnetic thin films and mesoscopic systems using a time resolved magneto-optical Kerr effect microscope (TR-MOKE). Reflected light from a magnetic material results in a rotation of the light polarization, effectively enabling the observation of magnetization precession. Our setup is based on a two-colour collinear pump-probe geometry. The Kerr rotation of the probe beam (λ = 1030 nm and pulse width ≈50 fs) is measured after exciting the sample with another laser beam of λ = 515 nm and a pulse width ≈50 fs. Both the pump and the probe beams are focused on the sample using a microscope objective, and a magnetic field can be applied at a small angle to the sample plane. The pump pulse rapidly modifies the out-of-plane demagnetizing field and thereby induces precessional magnetization dynamics. The precessional dynamics appears as an oscillatory signal on top of the decaying time-resolved Kerr rotation.
Magnetization Dynamics in Thin Films
We have characterized the magnetization dynamics of systems with two ferromagnetic thin films coupled antiferromagnetically across a non-magnetic layer shown schematically in Fig. 1a [1] and measured the spin wave dispersion on changing the alignment between the magnetization in the two layers from nearly antiparallel to parallel with an applied magnetic field. We observed a spin wave mode in addition to the expected acoustic and optical modes, which is a result of the laser-induced decoupling of the two ferromagnetic layers. A detailed description of how the applied magnetic field influences the magnetization dynamics is given in Ref [1], providing an important guide to the implementation of antiferromagnetically coupled films in functional spintronic devices.
In a second system with high perpendicular anisotropy, the magnetic damping is controlled by injecting spin currents that give rise to spin orbit torques [2]. Here, DC currents are sent to the film stack as show in Fig. 1b to generate the spin current. We find that the influence of the spin current on the damping is highly sensitive to the relative direction of the spin polarization and the precessional axis of the magnetization. This control of damping is important for the transfer of information via spin waves.
Magnetization Dynamics in Mesoscopic systems
We have characterized the magnetization dynamics occurring in two different mesoscopic systems. The first is an artificial spin ice in which the unit cell consists of three interacting horizontal and vertical nanomagnets as shown in Fig. 2a and b [3]. This system possesses a locally broken structural inversion symmetry, and we observe a rich spin wave spectrum as a result. We determine how the individual modes evolve on increasing the structure size, starting with building block structures containing only three parallel oriented nanomagnets. This work highlights the role of symmetry breaking in defining the mode profiles and is relevant for the realization of reconfigurable magnonic crystals based on artificial spin ices.
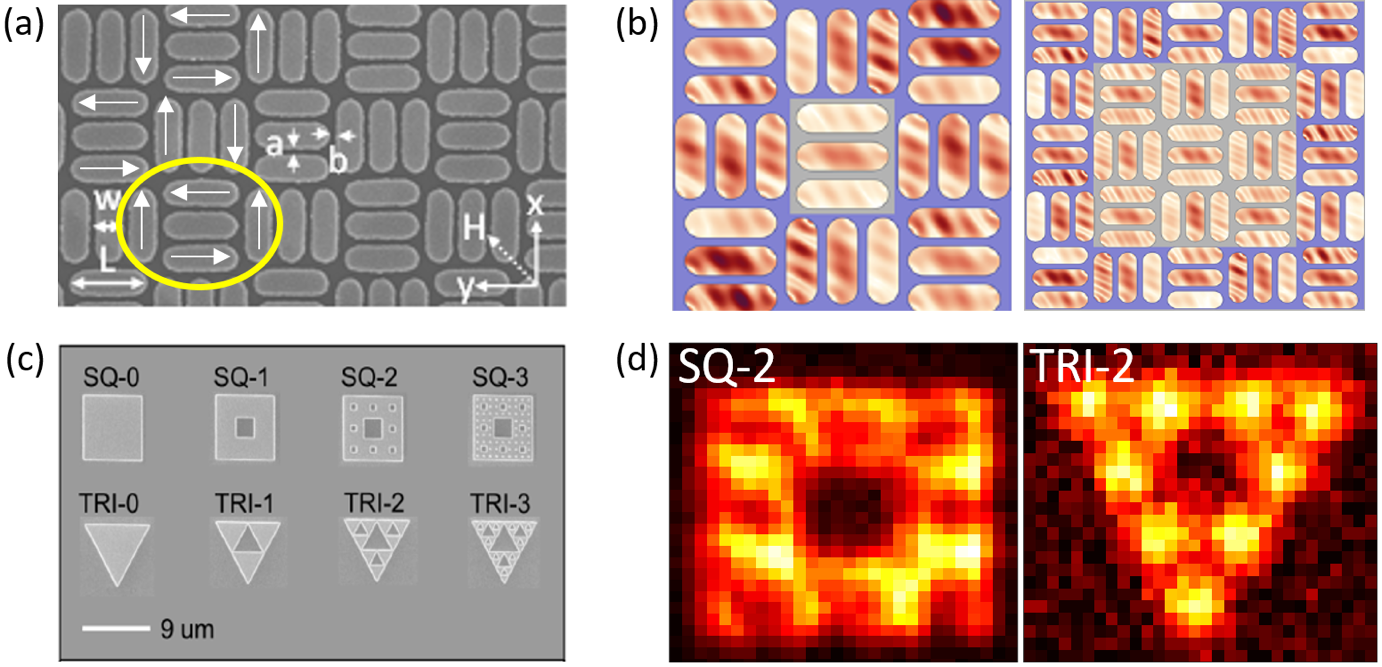
Another interesting set of magnetic structures that we characterized are based on fractals as shown in Fig. 2c and d [4]. Fractals consist of self-similar structures across different length scales, which look similar under different magnifications. We have investigated the magnetostatic mode formation in square and triangular fractal structures, where the mode formation is found to be related to the geometric scaling of the fractal structure.
References:
- Ultrafast-laser-induced magnetization dynamics in antiferromagnetically coupled ferromagnetic thin films
J. Zhou, S. Saha, Z. Luo, E. Kirk, V. Scagnoli and L. J. Heyderman, external page Phys. Rev. B, 101, 214434 (2020) - Control of Damping in Perpendicularly Magnetized Thin Films using Spin-Orbit Torques
S. Saha, P. Flauger, C. Abert, A. Hrabec, Z. Luo, J. Zhou, V. Scagnoli, D. Suess and L. J. Heyderman, external page Phys. Rev. B 101, 224401 (2020) - Spin-wave dynamics and symmetry breaking in an artificial spin ice
S Saha, J Zhou, K Hofhuis, A Kákay, V Scagnoli, LJ Heyderman, S Gliga, external page Nano Lett. 21, 2382 (2021) - Precessional dynamics of geometrically scaled magnetostatic spin waves in two-dimensional magnonic fractals
J. Zhou, M. Zelent, S. Perchenko, Z. Luo, E. Kirk, V. Scagnoli and M. Krawczyk, L. J. Heyderman, S. Saha, external page Phys. Rev. B 105, 174415 (2022)