Chiral nanomagnetic systems and logic
Magnetically coupled nanomagnets have many possible applications including non-volatile memories, logic gates, and sensors. So far, the most effective couplings have been found to occur between the magnetic layers in vertically layered stacks. We have exploited the Dzyaloshinskii-Moriya interaction to control the lateral coupling between out-of-plane and in-plane non-homogeneous magnetic structures, and demonstrate unique phenomena associated with such chirally coupled nanomagnets [1, 2]. Based on this chiral coupling, we have further develop a method of performing all-electric logic operations and cascading with domain-wall racetracks, providing a viable platform for novel logic-in-memory architectures [3, 4].
It is possible to design complex coupled nanomagnetic systems with lateral magnetic coupling such as those used for nanomagnet logic or artificial spin ice. Currently, most laterally coupled nanomagnet systems make use of the dipolar interaction, for which the coupling strength is proportional to the volume of nanomagnet. However, in order to electrically control these systems via spin-orbit torques, the nanomagnets should be extremely thin, which means that the dipolar coupling is very weak. Here, we couple the nanomagnets via the interfacial Dzyaloshinskii-Moriya interaction (iDMI). iDMI can be expressed by an energy term HDM = - Dij·(mi × mj), which represents the antisymmetric exchange coupling between two neighbouring magnetic moments mi and mj induced by the spin-orbit interaction in a structurally asymmetric environment (Fig. 1a). Since it is an interfacial effect, iDMI-induced coupling is only effective in thin magnetic films. In a homogeneous film, the presence of iDMI results in chiral domain walls and topological non-trivial magnetic textures such as cycloids or skyrmions.
We have patterned regions with in-plane (IP) and out-of-plane (OOP) magnetic anisotropy in a magnetic element using selective oxidation of Pt/Co/Al films (Fig. 1b). In addition to classical non-chiral interactions, the magnetization in the OOP and IP parts of the islands are coupled via iDMI arising from the Pt underlayer. As shown in Fig. 1b, this results in chirally coupled magnetization so that, when the IP region is set to point to the right (→) with an in-plane magnetic field, the OOP regions on the left and right of the IP region point down (⊗) and up (⊙) accordingly, giving either ⊗→ or →⊙ configurations. This agrees with the left-handed chirality generally expected from the negative sign of the iDMI in Pt/Co/AlOx and shown in the schematics in Fig. 1a.
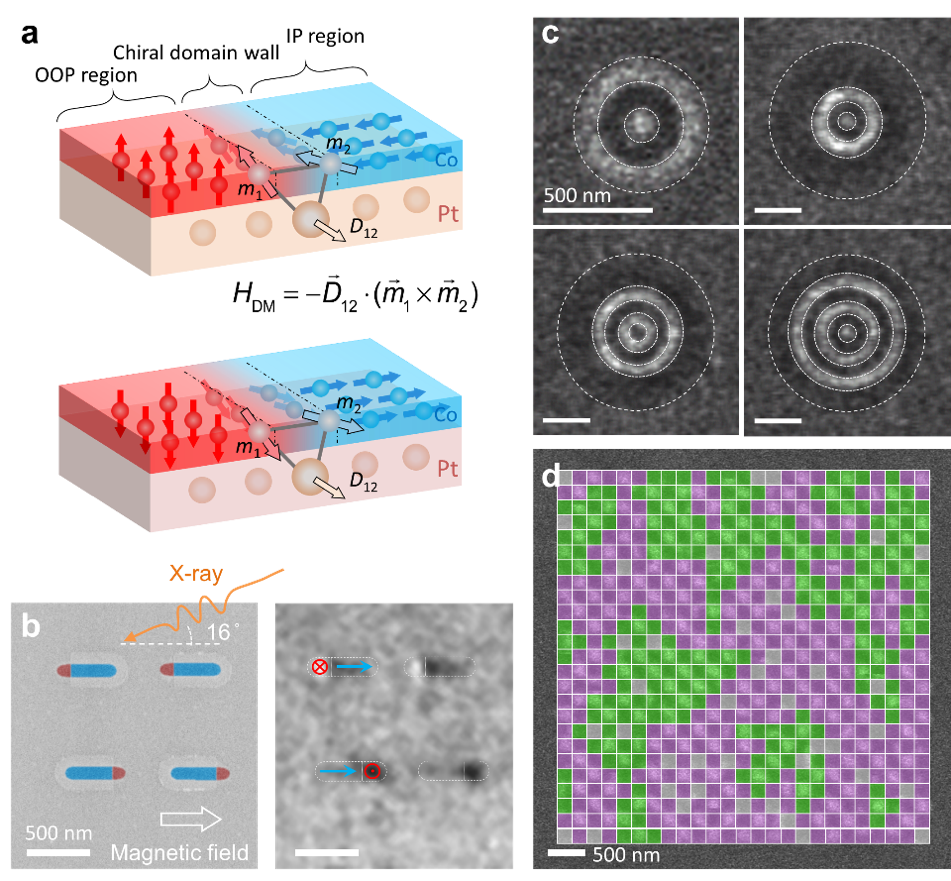
We have exploited this concept for various purposes in the single-domain regime such as lateral exchange bias, field-free current-induced switching between multistate magnetic configurations, as well as synthetic lateral antiferromagnets, skyrmions (Fig. 1c), and artificial spin ices (Fig. 1d) [1, 5, 6] covering a broad range of length scales. We have also developed this concept for device applications in the combination with mobile domain-walls (DWs) to realize chiral DW injection [7], to bias the DW torques [8] and to create DW logic devices [2, 3]. When passing a chiral DW through a narrow IP region, which acts as a logic inverter, an up|down (⊙|⊗) DW transforms into a ⊗|⊙ DW and vice versa (Fig. 2a). This inverter is equivalent to a NOT gate in Boolean logic with the binary data encoded into the magnetization of the moving domains, i.e. ⊗ =”1” and ⊙ =”0”. With devices based on this logic building block, we have demonstrated a complete set of all-electric logic operations and cascading with DW racetracks (Fig. 2b). The DW inverter can be also geometrically tailored to serve as a current-driven DW diode (Fig. 2c) [9]. The range of applications was also broadened with an investigation of the dynamic properties of polar-vortex oscillators established in chirally systems. Here we have shown that such current-driven oscillators can, as a result of their synchronization (Fig. 2d), serve as a platform for neuro-inspired computation [10]. With this work, we offer an alternative to go beyond the so-called “von Neumann bottleneck”, reducing the energy used and the time needed for the transfer of data between computation and storage units.
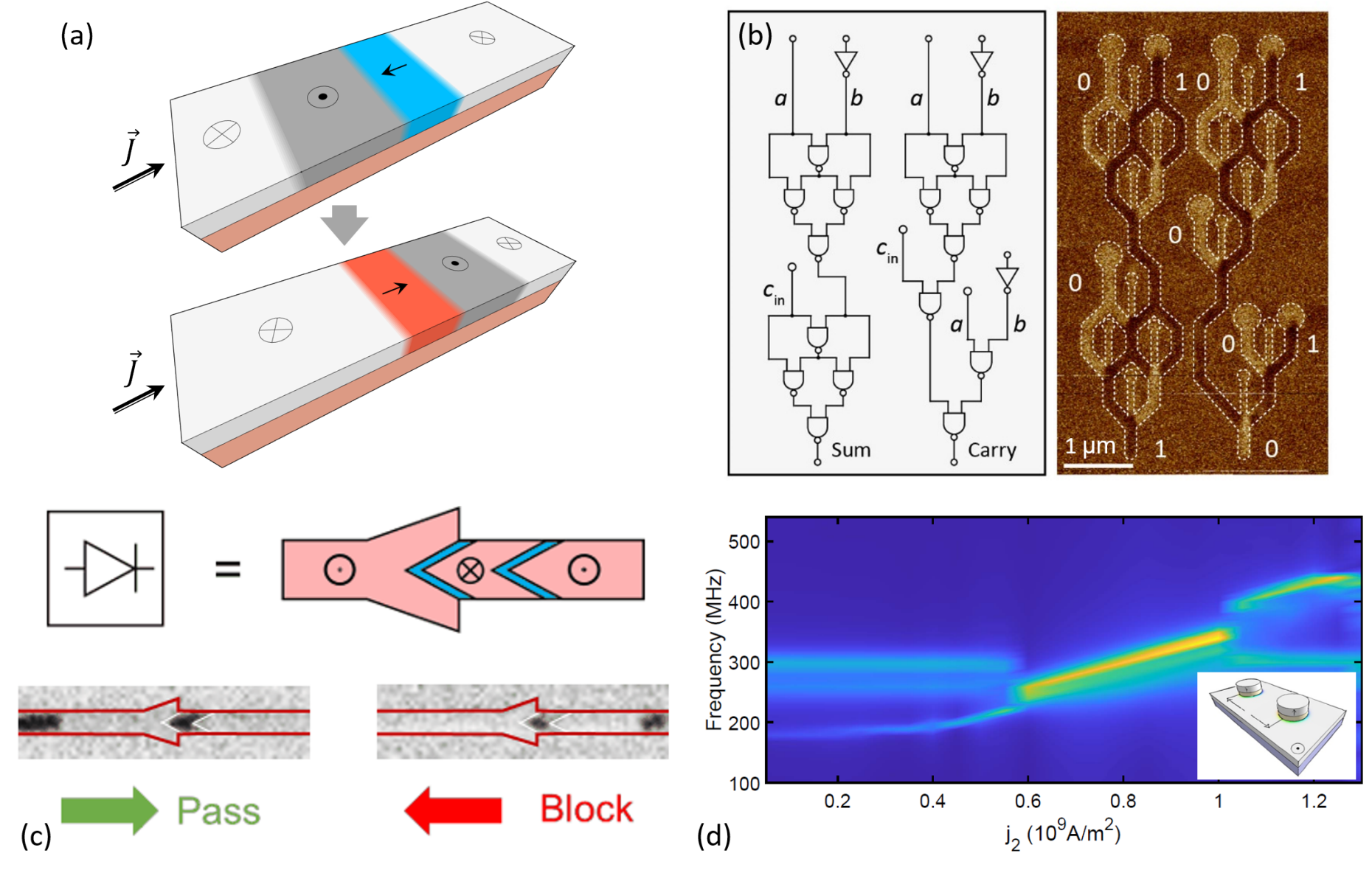
References:
1. Chirally coupled nanomagnets
Z. Luo, T. P. Dao, A. Hrabec, J. Vijayakumar, A. Kleibert, M. Baumgartner, E. Kirk, J. Cui, T. Savchenko, G. Krishnaswamy, L.J. Heyderman and P. Gambardella, external page Science 363, 1435 (2019).
2. Synthetic chiral magnets promoted by the Dzyaloshinskii–Moriya interaction
A. Hrabec, Z. Luo, L. J. Heyderman and P. Gambardella, external page Appl. Phys. Lett. 117, 130503 (2020)
3. Current-driven magnetic domain-wall logic
Z. Luo, A. Hrabec, T. P. Dao, G. Sala, S. Finizio, J. Feng, S. Mayr, J. Raabe, P. Gambardella and L. J. Heyderman, external page Nature 579, 214-218 (2020).
4. Current-driven magnetic domain wall logic
Z. Luo, A. Hrabec, T. P. Dao, L.J. Heyderman and P. Gambardella, European Patent EP20161352.8.
5. Artificial out-of-plane Ising antiferromagnet on the kagome lattice with very small further neighbour couplings
J. Colbois, K. Hofhuis, Z. Luo, X. Wang, A. Hrabec, L. J. Heyderman, F. Mila, external page Phys. Rev. B 104, 024418 (2021).
6. Geometrical control of disorder-induced magnetic domains in planar synthetic antiferromagnets
K. Hofhuis, X. Wang, A. Hrabec, Z. Luo, Z. Liu, P. Gambardella, P.M. Derlet, L.J. Heyderman, external page Phys. Rev. Mater. 6, L033001 (2022).
7. Chiral domain wall injector driven by spin–orbit torques
T. P. Dao, M. Müller, Z. Luo, M. Baumgartner, A. Hrabec, L. J. Heyderman and P. Gambardella, external page Nano Lett., 19, 5930-5937 (2019).
8. Engineering of Intrinsic Chiral Torques in Magnetic Thin Films Based on the Dzyaloshinskii-Moriya Interaction
Z. Liu, Z. Luo, S. Rohart, L. J. Heyderman, P. Gambardella, A. Hrabec, external page Phys. Rev. Appl. 16, 054049 (2021).
9. Field- and Current-Driven Magnetic Domain-Wall Inverter and Diode
Z. Luo, S. Schären, A. Hrabec, T. P. Dao, G. Sala, S. Finizio, J. Feng, S. Mayr, J. Raabe, P. Gambardella, and L. J. Heyderman, external page Phys. Rev. Applied 15, 034077 (2021)
10. Synchronization of chiral vortex nano-oscillators
Z. Zeng, Z. Luo, L. J. Heyderman, J.-V. Kim, A. Hrabec, external page Appl. Phys. Lett. 118, 222405 (2021).