Artificial Spin Ice - Phases & Phase Transitions
Artificial spin ice, that consists of arrays nanomagnets placed on two-dimensional lattices, is an example of a magnetic system that is frustrated as a result of the competition between the dipolar interactions [1, 2]. When the nanomagnets are superparamagnetic, the magnetization dynamics can be probed with a variety of techniques including x-ray photoemission electron microscopy, synchrotron x-ray scattering, muon spin spectroscopy and small angle neutron scattering. We are interested in using these techniques to observe phase transitions and the associated magnetic phases in artificial spin ice.
Artificial Square Ice
In initial experiments with synchrotron x-ray scattering, we observed the signature of long range ground-state ordering of the nanomagnet moments in artificial square ice [3]. We were also able to determine the number of reversed moments as a function of applied magnetic field for each of the two sublattices.
In later work on artificial square ice, we were able to establish the critical properties of the antiferromagnetic phase transition. Here we combined soft x-ray resonant magnetic scattering experiments with Monte Carlo simulations, and obtained quantitative evidence of a continuous magnetic phase transition with critical exponents that are consistent with those of the two-dimensional Ising universality class [4].
Artificial Kagome Spin Ice
The artificial kagome spin ice is predicted to undergo two phase transitions on decreasing the temperature. With low energy muon spectroscopy, we observed two peaks in the muon relaxation rate that can be identified with the critical temperatures of the predicted phase transitions [5]. Subsequently, on measuring the diffuse signal from x-ray scattering, we have observed the signature of the highest temperature Kagome Ice I phase, see Fig. 1 [6].
In an attempt to observe the phase transitions directly with x-ray photoemission electron microscopy, an interfacial Dzyaloshinskii-Moriya interaction was introduced to reduce the blocking temperature of the individual nanomagnets. This allowed us to observe the ground state in a seven-ring kagome structure [7].
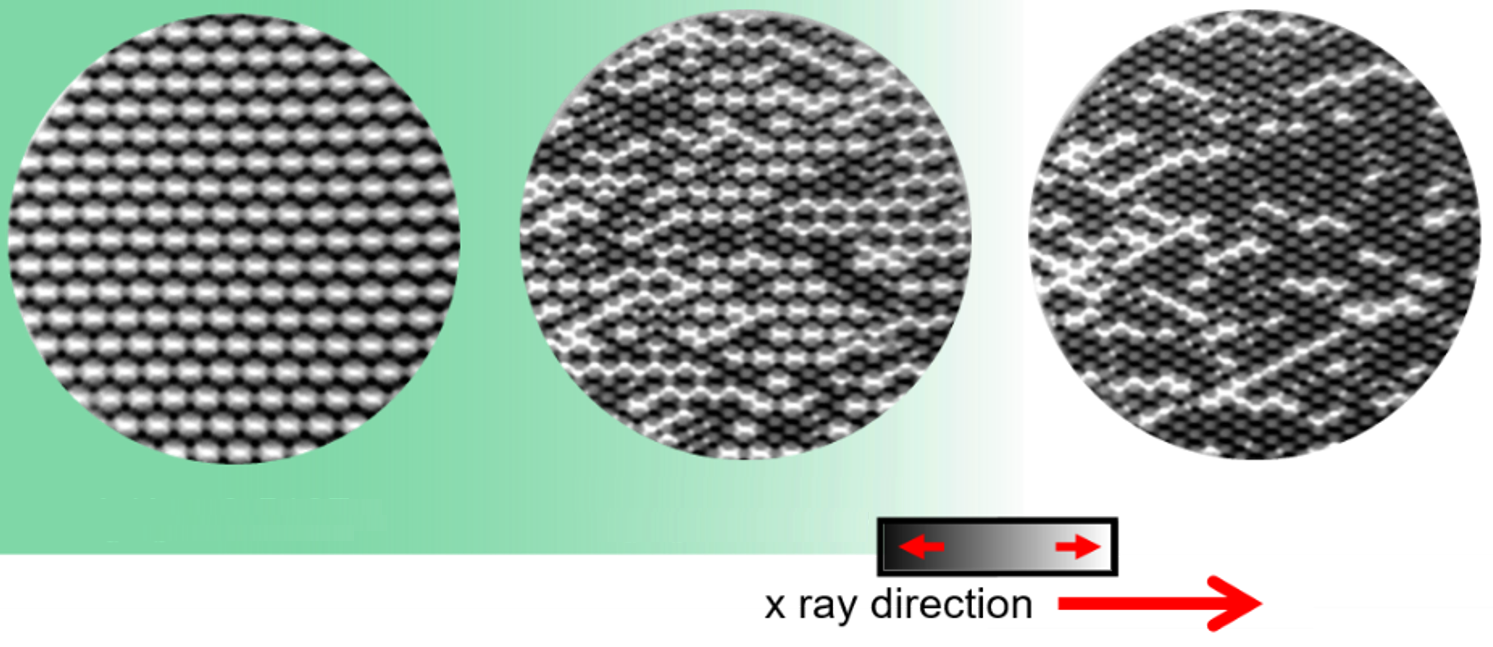
By introducing nanoscale bridges at the vertices where the nanomagnets meet, and with careful tuning of the interactions, it was possible to observe long range order in a bridged artificial kagome spin ice (see Fig. 2) as well as the evolution of the magnetic configurations associated with a phase transition in real space and time [8].
XY Systems
Using muon spin spectroscopy we have observed the phase transition in a system with dipolar coupled XY spins on the square lattice, see Fig. 3 [9]. In collaboration with the Condensed Matter Theory group at the Paul Scherrer Institute, it was possible to determine the phase diagram in the presence of disorder using state-of-the-art simulations and group theoretical descriptions [10].
Artificial triangular lattice Ising spin system
We have observed the magnetic correlations in an artificial triangular lattice Ising spin system using grazing-incidence small-angle neutron scattering [11]. By fitting a model structure factor to theoretical predictions of the frustrated triangular lattice, it was possible to correlate the theory with experiment, and the presence of a weakly correlated paramagnetic phase was established.
References
- Artificial ferroic systems: novel functionality from structure, interactions and dynamics
L.J. Heyderman and R.L. Stamps, external page J. Phys.: Condens. Matter 25, 363201 (2013) - Advances in Artificial Spin Ice
S.H. Skjærvø, C.H. Marrows, R.L. Stamps, L.J. Heyderman, external page Nature Reviews Physics 2, 13 (2020) - Extended reciprocal space observation of artificial spin ice with x-ray magnetic resonant scattering
J. Perron, L. Anghinolfi, B. Tudu, N. Jaouen, J.-M. Tonnerre, M. Sacchi, F. Nolting, J. Lüning, L.J. Heyderman, external page Phys. Rev. B 88, 214424 (2013) - Continuous magnetic phase transition in artificial square ice
O. Sendetskyi, V. Scagnoli, N. Leo, L. Anghinolfi, A. Alberca, J. Lüning, U. Staub, P.M. Derlet, L.J. Heyderman, external page Phys. Rev. B 99, 214430 (2019) - Thermodynamic phase transitions in a frustrated magnetic metamaterial
L. Anghinolfi, H. Luetkens, J. Perron, M.G. Flokstra, O. Sendetskyi, A. Suter, T. Prokscha, P.M. Derlet, S.L. Lee, L.J. Heyderman, external page Nat. Commun. 6, 8278 (2015) - Magnetic diffuse scattering in artificial kagome spin ice
O. Sendetskyi, L. Anghinolfi, V. Scagnoli, G.Möller, N. Leo, A. Alberca, J. Kohlbrecher, J. Lüning, L.J. Heyderman, external page Phys. Rev. B 93, 224413 (2016) - Thermally superactive artificial kagome spin ice structures obtained with the interfacial Dzyaloshinskii-Moriya interaction
K. Hofhuis, A. Hrabec, H. Arava, N. Leo, Y-L Huang, R.V. Chopdekar, S. Parchenko, A. Kleibert, S. Koraltan, C. Abert, C. Vogler, D. Suess, P.M. Derlet, L.J. Heyderman, external page Phys. Rev. B 102, 180405(R) (2020) - Real-space imaging of phase transitions in bridged artificial kagome spin ice
K. Hofhuis, S. Skjærvø, S. Parchenko, H. Arava, Z. Luo, A. Kleibert, P.M. Derlet, L.J. Heyderman, external page Nature Physics (2022)
- Collective magnetism in an artificial 2D XY spin system
N. Leo, S. Holenstein, D. Schildknecht, O. Sendetskyi, H. Luetkens, P. M. Derlet, V. Scagnoli, D. Lancon, J. R. L. Mardegan, T. Prokscha, A. Suter, Z. Salman, S. Lee, L. J. Heyderman, external page Nat. Commun. 9, 2850 (2018) - Phase diagram of dipolar-coupled XY moments on disordered square lattices
D. Schildknecht, L. J. Heyderman, P. M. Derlet, external page Phys. Rev. B 98, 064420 (2018) - Direct observation of spin correlations in an artificial triangular lattice Ising spin system with grazing-incidence small-angle neutron scattering
P. Pip, A. Glavic, S.H. Skjærvø, A. Weber, A. Smerald, K. Zhernenkov, N. Leo, F. Mila, L. Philippe, L.J. Heyderman, external page Nanoscale Horizons 6, 474 (2021)