Artificial Spin Ice - Slow Dynamics
Artificial spin ice, which consist of arrays of dipolar coupled single-domain nanomagnets arranged in particular geometries, have drawn increasing interest from the research community as they provide the possibility to investigate the effects of geometrical frustration in real space and time [1,2]. Manufactured with electron beam lithography, one can fine tune the structure of the system in terms of nanomagnet size, lattice geometry and lattice periodicity. The system behaviour in response to an external stimulus can then be investigated.
Over the past years, much of the research has been directed towards investigations of two geometries, namely artificial square ice and artificial kagome spin ice. Artificial square ice was first introduced as a two-dimensional analogue to pyrochlore spin ice, where the role of the Ising spins is mimicked by the magnetic moments of elongated nanomagnets, whose shape anisotropy fixes their moment direction.
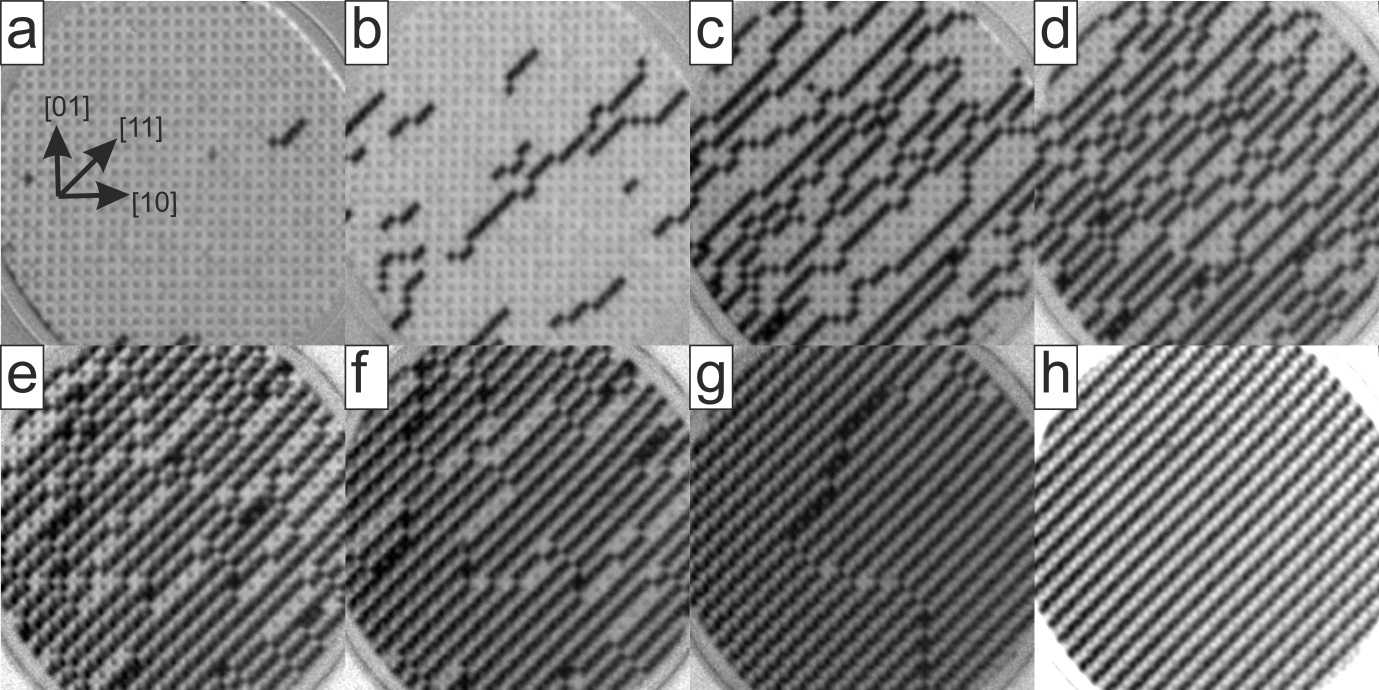
Our initial research on artificial spin ice concentrated on field-driven experiments [3-5]. Employing x-ray photoemission electron microscopy (PEEM) to resolve the exact arrangement of the nanomagnet moments, we investigated the possibility to achieve with demagnetization procedures the low-energy configurations in the building blocks of artificial kagome ice [3,4], and we observed the creation and propagation of emergent magnetic monopoles in extended arrays [5].
Subsequently we fabricated artificial spin ice structures that exhibit fluctuating magnetic moments at experimentally accessible temperatures (see Fig. 2). This was achieved either by patterning Permalloy (Ni80Fe20) wedge films [6,7] or 𝛿-doped Pd(Fe) films in collaboration with the group of Björgvin Hjovarrson at Uppsala University [8]. In artificial square ice, we were able to follow in real time a thermally-driven relaxation process from an initial high energy configuration of the magnetic moments to a ground state configuration [6] (see Fig. 1). In building blocks of artificial kagome ice, our simple thermal annealing procedure, that involves a moderate heating above the nanomagnet blocking temperatures (TB = 320-330 K), proved to be highly effective in achieving the low-energy configurations [7]. However, with increasing systems size, it becomes more and more difficult to access the predicted ground state configurations.
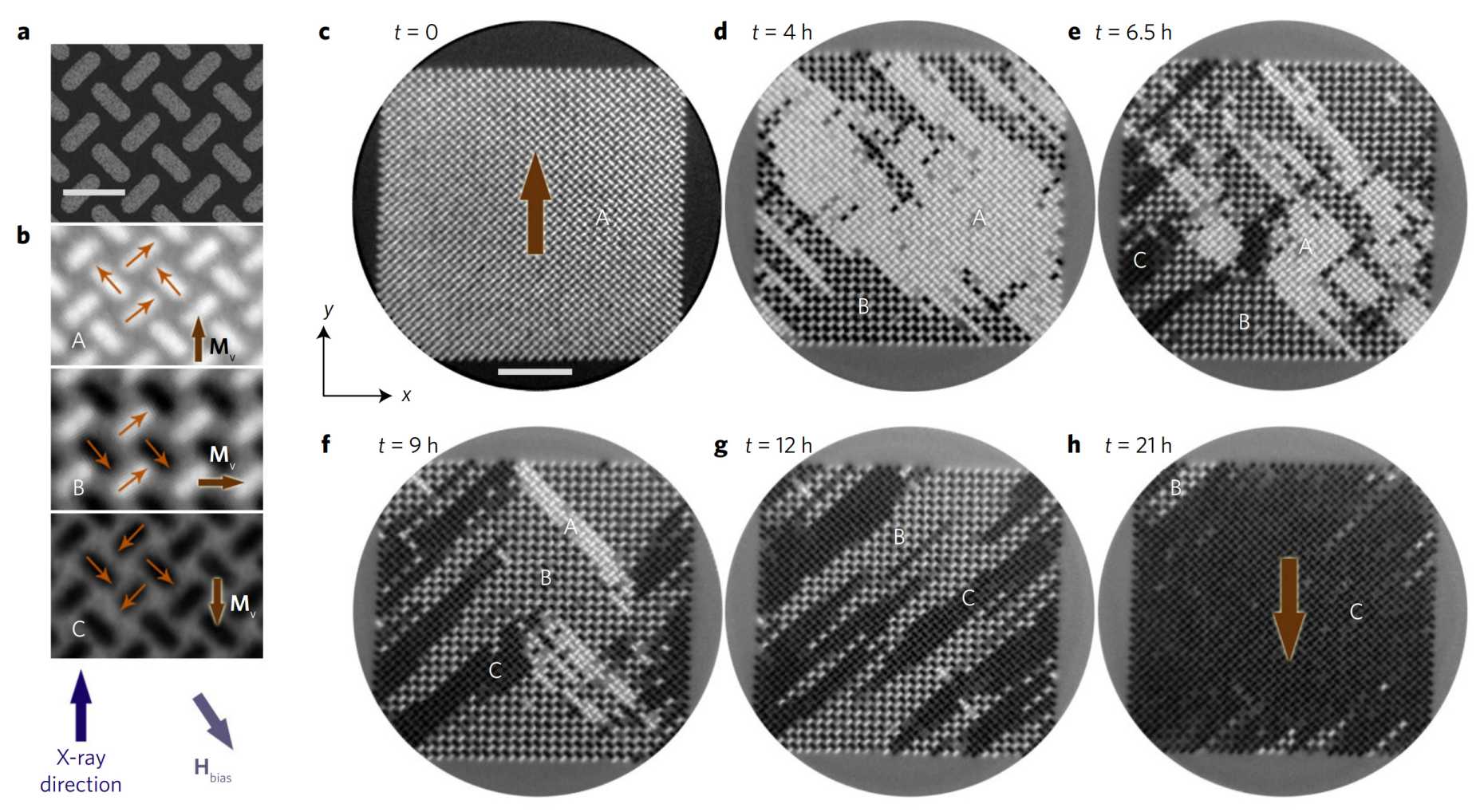
We have also investigated an artificial spin ice system with a modified geometry, derived from the square ice [9]. Employing PEEM to measure the evolution of a thermally-active system at room temperature, we have established that the average magnetization displays chiral dynamics: it consistently rotates in a unique direction following saturation with an external field (see Fig. 3). Here, the topology of the magnetostatic interactions effectively creates a collective ratchet-like behaviour. This implementation of a 'chiral ice', demonstrates how artificial spin ice can be tailored into a functional material, which converts energy into a well-defined rotation of the magnetization. The fact that this system behaves like a ratchet opens the possibility of using artificial spin ice in nanoscale devices such as nanomotors, actuators or sensors.
In a collaboration with the Laboratory for Multifunctional Ferroic Materials, we have modified a square ice structure so that every nanomagnet in the array is replaced by two nanomagnets. Such an artificial magneto-toroidal crystal can be poled with a magnetic tip [10] and, by altering the geometry, can give insight into how the intermagnet interactions influence the magnetic domain structure [11],
References:
- Artificial ferroic systems: novel functionality from structure, interactions and dynamics
L.J. Heyderman and R. L. Stamps, external page J. Phys.: Condens. Matter 25, 363201 (2013) - Advances in Artificial Spin Ice
S.H. Skjærvø, C.H. Marrows, R.L. Stamps, L.J. Heyderman, external page Nature Reviews Physics 2, 13 (2020) - Building blocks of an artificial kagome spin ice: Photoemission electron microscopy of arrays of ferromagnetic islands
E. Mengotti, L.J. Heyderman, A. Fraile Rodriguez, A. Bisig, L. Le Guyader, F. Nolting, H.B. Braun, external page Phys. Rev. B 78, 144402 (2008) - Controlling vortex chirality in hexagonal building blocks of artificial spin ice
R.V. Chopdekar, G. Duff, R.V. Hugli, E. Mengotti, D.A. Zanin, L.J. Heyderman, H. B. Braun, external page New J. Phys. 15, 125033 (2013) - Real-space observation of emergent magnetic monopoles and associated Dirac strings in artificial kagome spin ice
E. Mengotti, L.J. Heyderman, A. F. Rodriguez, F. Nolting, R.V. Hugli, H.B. Braun, external page Nat. Phys. 7, 68-74 (2011) - Direct Observation of Thermal Relaxation in Artificial Spin Ice
A. Farhan, P.M. Derlet, A. Kleibert, A. Balan, R.V. Chopdekar, M. Wyss, J. Perron, A. Scholl, F. Nolting, L.J. Heyderman, external page Phys. Rev. Lett. 111, 057204 (2013) - Exploring hyper-cubic energy landscapes in thermally active finite artificial spin-ice systems
A. Farhan, P.M. Derlet, A. Kleibert, A. Balan, R.V. Chopdekar, M. Wyss, L. Anghinolfi, F. Nolting, L.J. Heyderman, external page Nat. Phys. 9, 375-382 (2013) - Thermal fluctuations in artificial spin ice
V. Kapaklis, U.B. Arnalds, A. Farhan, R.V. Chopdekar, A. Balan, A. Scholl, L.J. Heyderman, B. Hjörvarsson, external page Nature Nanotechnology 9, 514 (2014)
- Emergent dynamic chirality in a thermally driven artificial spin ratchet
S. Gliga, G. Hrkac , C. Donnelly, J. Büchi, A. Kleibert, J. Cui , A. Farhan, E. Kirk, R. V. Chopdekar, Y. Masaki, N. S. Bingham, A.Scholl, R. L. Stamps, L. J. Heyderman, external page Nat. Mater. 16,1106–1111(2017) - Poling of an artificial magneto-toroidal crystal
J. Lehmann, C. Donnelly, P.M. Derlet, L.J. Heyderman, M. Fiebig, external page Nature Nanotechnology 14, 141 (2019)
external page - Relation between microscopic interactions and macroscopic properties in ferroics
J. Lehmann, A. Bortis, P.M. Derlet, C. Donnelly, N. Leo, L.J. Heyderman, M. Fiebig, external page Nature Nanotechnology 2020, 15, 896 (2020)